
- This event has passed.
Advances in modelling defects and interfaces workshop
14 October @ 1:30 pm – 15 October @ 2:00 pm
Institute of Physics (IOP), London
The Thomas Young Centre takes great pleasure to announce the workshop “Advances in modelling defects in solids and interfaces”, being organised to honour the achievements of Professor Alexander Shluger in modelling defects and interfaces in solids and nanosystems and contributions to computational materials science.
Alex has been part of the UK Computational Materials Science community for more than 30 years, first working at the Royal Institution, and since 1996 at University College London, where he has been a Head of the Condensed Matter and Materials Physics group and co-director of the Thomas Young Centre.
He has made important contributions to the theoretical modelling of defects in the bulk and at surfaces and interfaces of insulators. He also contributed to developing models explaining mechanisms of imaging and manipulation of surface atoms and molecules using atomic force microscopy. His achievements were recognised by the Institute of Physics (IoP) by the award of the David Tabor Medal and Prize in 2020.
Alex continues to maintain a very active research group at UCL: https://www.ucl.ac.uk/condensed-matter-material-physics/alexander-shluger-group
Monday 14 October
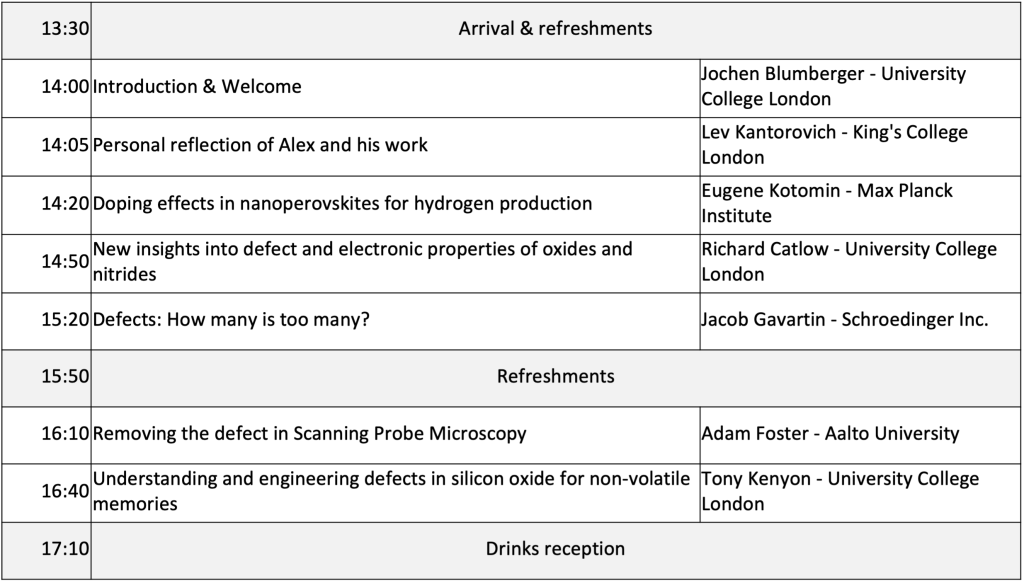
Tuesday 15 October
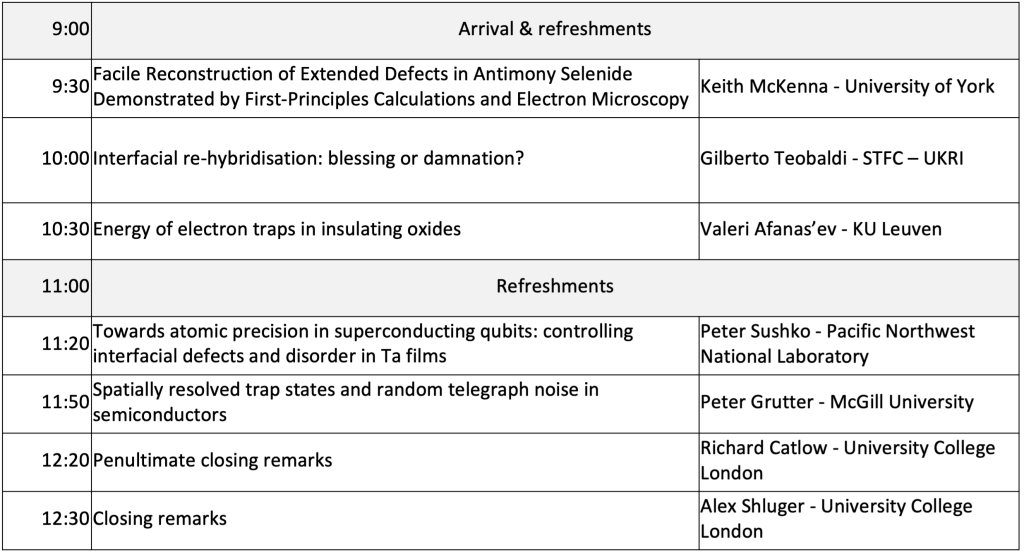
10 invited speakers will deliver research talks at the forefront of computational materials science, who are long-term collaborators or former students and postdocs of Alex:
Energy of electron traps in insulating oxides – Valeri Afanas’ev, KU Leuven, Belgium
For more than 60 years insulating oxides were the key enables of integrated semiconductor electronics evolving from the “classical” SiO2 thermally grown on Si to the nowadays successors like high-k (Al2O3, HfO2, ZrO2,…) and low-k (porous SiOCN matrices) layers. Not surprisingly, reliability of these insulators became the core issue in securing sufficient lifetime of the functional devices. Charge trapping, development of leakage current and, as the ultimate failure, dielectric breakdown are seen as the consequence of injected electronic charges prompting a deeper understanding of electron-network interactions. Though in the case of SiO2 the decennia of research by electron spin resonance (ESR) revealed significant impact of dangling bond (DB) defects (Pb-, E’-type centers), it appears not to be the case in high-k metal oxides urging to re-consider the DB paradigm as whole. In these systems, the ESR analysis was mostly successful in revealing the impurity-related trapping sites but not the intrinsic ones. In these circumstances, the spectroscopic information about critically important imperfections of the oxide matrix should be delivered by alternative methods. The most straightforward approach addresses the core effect – charges generated due to electron and hole trapping. Since microscopic parameters of the trapping process (capture cross section, trapping rate, etc.) do not deliver information about atomic arrangements responsible for the trapping, one might consider analysis of the “final result”, i.e., the trapped electron (hole) state, to “recognize the enemy”. It will be shown that, unlike in the case of SiO2, the trapped electron sites in high-k metal oxides can be assessed by optical excitation (de-population, i.e., the trapped charge removal) which opens the way to their spectroscopic identification through trapped electrons energy distribution. Several typical energies of the trapped electrons can be identified within the bandgap of insulating oxides. With this information as the solid reference, theoretical modelling of the defects emerges an unmissable tool of defect identification. As the oxygen deficiency models appear to fail in explaining the electron trapping evolution as affected by technological processing, one is prompted to search for alternative culprits for electron trapping. The simulations reveal that the experimentally observed deep (2-3 eV) electron trapping can be explained by self-localization of electrons in polaronic states related to disorder of the amorphous oxide network. Furthermore, comparison of optical and thermal energies of electron escape from the trap allowed us to estimate the relaxation energy which also appears to be consistent with the polaronic hypothesis. These results show that, even in the absence of atomic information delivered by ESR, the trapping sites can be identified on the basis of their energy spectrum using modelling of the trapping sites.
New insights into defect and electronic properties of oxides and nitrides – Richard Catlow; Department of Chemistry, University College London
We will discuss recent work on the defect and electronic structure of technological important oxide and nitride materials. We will highlight the role of QM/MM methods in modelling these materials and will show how these methods can be integrated with other computational approaches. Recent work on the following materials will be reviewed:
- CeO2 where we will show how by applying QM/MM techniques in conjunction with Mott-Littleton and periodic methods, we have developed a consistent set of models for the defect and electronic structure of the material.
- AlN and GaN, where we characterise the basic defect structure and in the latter case discuss dopant-defect complexes leading to p-type conductivity
- ZnO where we discuss both the intrinsic defect structure and that of Li and Cu doped systems.
Whole focusing on these materials, we will aim to give a broader perspective on modelling defects in insulators and semiconductors.
Removing the defect in Scanning Probe Microscopy – Adam Foster, Aalto University, Finland
Scanning Probe Microscopy (SPM) has been the engine of characterization in nanoscale systems in general, and the evolution of functionalized tips as a reliable tool for high-resolution imaging without material restrictions has been a breakthrough in studies of molecular systems [1]. In parallel, machine learning (ML) methods are increasingly being applied to data challenges in SPM. In particular, the success of deep learning in image recognition tasks has led to their application to the analysis of SPM images, especially in the context of surface feature characterisation and techniques for autonomously-driven SPM [2].
In this work, we explore the general potential for using ML approaches to aid in the analysis of Atomic Force Microscopy (AFM) and Scanning Tunnelling Microscopy (STM) images, along with the possibilities of introducing ML automation into experimental workflows. As an example, we build upon a deep learning infrastructure that matches a set of AFM/STM images with a unique descriptor characterizing the molecular configuration [3], and then develop a workflow that takes experimental images of complex molecular systems and revises initial ML structure predictions with neural network potential simulations [4]. In this context, we discuss the challenges of handling experimental data and possible data augmentation strategies. Beyond this, we show how ML approaches can be used actively during SPM experiments for construction of nanostructures through atomic manipulation [5], while also highlighting approaches towards automated construction of more complex molecular systems atom-by-atom and bond-by-bond.
References:
[1] N. Pavliček and Leo Gross, Nature Reviews Chemistry 1, 1–11 (2017)
[2] O.M. Gordon and P.J. Moriarty, Mach. Learn.: Sci. Technol. 1 (2020) 023001; Sergei V. Kalinin et al, MRS Bulletin (2022) s43577-022-00413-3
[3] B. Alldritt, P. Hapala, N. Oinonen, F. Urtev, O. Krejci, F. F. Canova, J. Kannala, F. Schulz, P. Liljeroth, and A. S. Foster, Sci. Adv. 6 (2020) eaay6913; Lauri Kurki, Niko Oinonen and Adam S. Foster, ACS Nano (2024) acsnano.3c12654
[4] F. Priante, N. Oinonen, Y. Tian, D. Guan, C. Xu, S. Cai, P. Liljeroth, Y. Jiang, and A. S. Foster, ACS Nano (2024) acsnano.3c10958
[5] I-Ju Chen, Markus Aapro, Abraham Kipnis, Alexander Ilin, Peter Liljeroth and Adam S. Foster, Nat. Commun. 13 (2022) 7499
Defects: How many is too many? – Jacob Gavartin, Schroedinger Inc.
Solid state defects are typically defined as a 1,2, or 3-dimensional violation of an otherwise “perfect” crystal atomic order. It is a powerful concept which has been tremendously successful in the description of numerous properties of real materials. The progress in theory of defects has been significant, not least due to the pioneering contributions of UCL academicians such as professors Marshall Stoneham, Richard Catlow and Alex Shluger to name a few. The success in this field has led to a change of current theoretical aspirations from descriptive models to quantitative predictions of defect related properties. In this contribution I shall discuss a few examples where a canonical notion of a defect as an isolated species breaks down due to the defects’ interactions, so that defect aggregated properties differ significantly from those of isolated defects. The discussed phenomena include:
- Charged defects and charge compensation mechanisms
- Defects concentration prediction
- Defects agglomeration and segregation effects and phase transitions
- Defects kinetics versus thermodynamics
Based on these examples I shall address successes and challenges of the reliable predictions by atomistic modelling.
Spatially resolved trap states and random telegraph noise in semiconductors – Peter Grutter, McGill University, Canada
Semiconductor interfaces often have isolated trap states which modify electronic properties. Here, we study the electric susceptibility of the Si/SiO2 interface with nm spatial resolution using frequency-modulated atomic force microscopy. We show that surface charge organization timescales, which range from 1−150 ns, increase significantly around interfacial states [1]. We conclude that dielectric loss under time-varying gate biases at MHz and sub-MHz frequencies in metal-insulator-semiconductor capacitor device architectures is highly spatially heterogeneous over nm length scales [1].
In frequency-modulated atomic force microscopy the measured frequency shift is quadratic in applied bias for metallic samples and probes. However, for semiconducting samples, band bending effects must be considered, resulting in non-parabolic bias curves. We have developed a framework to quantitatively describe a metal-insulator semiconductor (MIS) device formed out of a metallic AFM tip, vacuum gap, and semiconducting sample. We show how this framework allows us to measure dopant concentration, bandgap and band bending timescales of different types of defects on semiconductors with nm scale resolution on Si, 2D MoSe2 and pentacene monolayers [2].
We also measure temporal two-state fluctuations of individual defects at the Si/SiO2 interface with nanometer spatial resolution using frequency-modulated atomic force microscopy with single electron sensitivity. We demonstrate that two-state fluctuations are localized at interfacial traps, with bias-dependent rates and amplitudes. When measured as an ensemble, the observed defects have a 1/f power spectral trend at low frequencies [3].
Low-frequency noise due to two level fluctuations inhibits the reliability and performance of nanoscale semiconductor devices, and challenges the scaling of emerging spin based quantum sensors and computers. The presented method and insights provide a more detailed understanding of the origins of 1/f noise in silicon-based classical and quantum devices, and could be used to develop processing techniques to reduce two-state fluctuations associated with defects.
Understanding and engineering defects in silicon oxide for non-volatile memories – Tony Kenyon, University College London, UK
Silicon oxide, for many years regarded as a stable dielectric and widely deployed in CMOS electronics, can, by careful defect engineering, be transformed into an excellent material for resistance switching. In this talk I will discuss its application in resistive RAM and memristive devices, highlighting how important it is to combine atomistic modelling with experimental work to understand and engineer the contribution of bulk and interface defects.
Doping effects in nanoperovskites for hydrogen production – Eugene Kotomin, Max Planck Institutes, Germany
Hydrogen production directly from water is the efficient source for green, environmentally friendly energy. Sunlight-driven water splitting is one of the most promising pollution-free strategies for production of hydrogen. Photocatalytic water splitting consists of water decomposition into hydrogen and oxygen by a reaction with photo-generated charge carriers. However, many challenges must be overcome before photocatalytic water splitting can be practically implemented at a large scale. We discuss the results of large scale first-principles calculations on structural and electronic properties of SrTiO3 (STO) perovskite photocatalyst (band gap 3.25 eV) and how to modify its electronic band structure by means of defects and impurities. DFT calculations were performed with CRYSTAL17 computer code within the linear combination of atomic orbitals (LCAO) approximation and using B1WC advanced hybrid exchange-correlation functional. We considered the bulk STO crystal and its (001) 2D slabs, as well as faceted nanoparticles. A supercell was used to simulate point defects (neutral and charged oxygen vacancies, N and Al substitutional atoms [1-4]). Introduction of these defects indeed makes STO photocatalyst more efficient for sunlight-driven water splitting.
Facile Reconstruction of Extended Defects in Antimony Selenide Demonstrated by First-Principles Calculations and Electron Microscopy – Keith McKenna, University of York, UK
Most crystalline materials, whether naturally occurring or manufactured for technology, are polycrystalline, making grain boundaries one of the most ubiquitous types of structural defect. Grain boundaries in semiconductors and insulators often cause significant modification of electronic, optical and transport properties, therefore affecting the performance of polycrystalline materials for diverse technologies (e.g., in photochemistry, photovoltaics, energy storage and electronics). Computational modelling of grain boundaries can bring valuable insights into fundamental properties and aid in the discovery and optimisation of materials for applications.
In the first part of this talk, I will provide a brief introduction to computational approaches for modelling grain boundaries, providing examples from our previous work spanning a range of materials. Through these examples the power of combining first-principles calculations and electron microscopy for understanding grain boundaries will be highlighted [1].
In the second part of the talk, I will present our investigations into the structure and properties of extended defects in antimony selenide (Sb2Se3) and related semiconductors that are promising for application as solar absorbers in thin-film photovoltaic and photoelectrochemical cells [2,3]. Different from the conventional picture that emerges from studies of many other compound semiconductors, we show that dangling bonds introduced at such extended defects such as surfaces and grain boundaries readily reconstruct to eliminate deleterious deep gap states associated with enhanced electron-hole recombination [4,5]. Our calculations predict the reconstruction of extended defects leads to significant long-range strain fields which have subsequently been observed using scanning transmission electron microscopy [6]. Preliminary results for structurally-similar chalcohalide materials indicate this behaviour may be common to a wider range of promising semiconductors conferring some degree of intrinsic grain boundary tolerance.
References
[1] J. Quirk et al, Appl. Phys. Rev. 11, 011308 (2024).
[2] Y. Zhao et al, Adv. Energy Mater. 12, 2103015 (2022).
[3] Z. Duan et al, Adv. Energy Mater. 34, 2202969 (2022).
[4] R.E. Williams et al, ACS Appl. Mater. & Inter. 12, 21730 (2020).
[5] K.P. McKenna, Adv. Electron. Mater. 7, 2000908 (2021).
[6] R.A. Lomas-Zapata et al, Phys. Rev. X Energy 3, 013006 (2024).
Towards atomic precision in superconducting qubits: controlling interfacial defects and disorder in Ta films – Peter Sushko, Pacific Northwest National Laboratory, USA
In recent years, there has been significant progress in the development of platforms for quantum computing. A breakthrough in quantum computing hardware has been the discovery of superconducting quantum circuits, which offer scalability and low error rates. However, the practical implementation of superconducting qubits in a quantum processor is hindered by their limited coherence lifetime.
Coherence times of transmon devices can be affected by oxidation of the components made of superconducting metals, such as Nb and Ta. Spontaneous oxidation results in the formation of suboxide phases and surface amorphization that contribute to dielectric losses that are primarily attributed to two-level systems within such native oxide layers. Mitigating undesirable effects of surface oxidation requires understanding the mechanisms of interfacial interactions at the atomic scale.
We will review recent experimental studies that provide new insights into the atomic structure and composition of the native oxide layer and focus on ab initio simulations of the mechanisms of Ta and Nb interaction with oxygen. In particular, we consider factors controlling the early stages of Ta film growth, and energetics and pathways of the Ta film oxidation, including propagation of the oxidation front into the Ta subsurface and corresponding electronic structure changes, and explore strategies for suppressing Ta oxidation using reactive metal coatings. We will also consider the mechanism of defect accumulation at the metal/metal oxide interfaces and discuss models of candidate two-level systems.
C. Zhou, J. Mun, J. Yao, A. K. Anbalagan, M. D. Hossain, R. A. McLellan, R. Li, K. Kisslinger, X. Tong, G. Li, A. R. Head, C. Weiland, A. L. Walter, Q. Li, Y. Zhu, P. V. Sushko, M. Liu, Ultrathin magnesium-based coating as an efficient oxygen barrier for superconducting circuit materials, Advanced Materials 36, 2310280 (2024). DOI: 10.1002/adma.202310280
J. Mun, P. V. Sushko, E. Brass, C. Zhou, K. Kisslinger, X. Qu, M. Liu, Y. Zhu, Probing oxidation-driven amorphized surfaces in a Ta(110) film for superconducting qubit, ACS Nano 18, 1126-1136 (2024). DOI: 10.1021/acsnano.3c10740
Interfacial re-hybridisation: blessing or damnation? – Gilberto Teobaldi, STFC – UKRI
Interfacial electronic re-hybridisation (ER), and the ensuing emergence of properties different from the isolated interface-constituents, has long attracted scientific and technological interest. Understanding ER holds the key to control interfacial properties, and promote rational advances in those technologies whose functioning (or failure) rests on contacting different materials. Control of ER may also enable the definition of new solutions by interfacing expectedly unappealing, yet readily available, materials. The residual challenges in atomic and time resolved experimental characterisation of interfaces make Density Functional Theory (DFT) a valuable source of atomistic insights, albeit with intrinsic accuracy-viability compromises. DFT can also be used to inexpensively explore materials and strategies to tailor interfacial ER and emergent properties for a given application. Along these lines, here I will present an overview of recent results, insights and, where available experimental validation, on the potential of interfacial ER for applications as diverse as enhancement of magnetism in transition-metals, stabilisation of alkali-metal anodes for high energy-density batteries, and control of redox kinetics at photo/electro-chemical interfaces.
We expect an audience of scientific peers in computational materials research, from PhD students to Senior Professors.
Please direct any queries you have to Karen Stoneham (tyc-administrator@ucl.ac.uk).
