
- This event has passed.
TYC Inaugural Lecture: Thomas Keal – Scaling up computational chemistry: from small molecules to complex systems
27 April @ 2:00 pm – 5:00 pm
Venue: Ramsay Lecture Theatre, followed by a reception in the Nyholm Room, Christopher Ingold Building
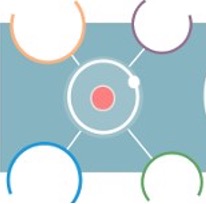
14:00 Michael Buehl, St Andrews – Enzymology in silico
Enzymes with their well-defined active sites are targets for QM/MM applications par excellence. Two case studies of such applications will be discussed, where insights into enzyme function have been obtained from DFT/Charmm calculations. The first one is on the origin of the oxidative power of ligninolytic enzymes, believed to be at the heart of their ability to degrade lignin.1 Trends in redox potentials across a series of heme-based peroxidases (Figure 1a), as well as their high sensitivity to pH, are well captured computationally, but contrary to earlier proposals,1a no simple rationalisation of these findings is emerging.1b The second case study involves explores the reaction mechanism of Is-PETase, a recently discovered enzyme capable of degrading PET. Surprisingly low activation barriers for serine protease-type hydrolysis steps are computed (Figure 1b),2 suggesting that other steps, notably substrate binding and/or product release, to be rate lomiting.
References
1. a) L. Castro, L. Crawford, A. Mutengwa, J. Götze, M. Bühl, Org. Biomol. Chem. 2016, 14, 2385; b) J. D. Colburn, M. Bühl, unpublished.
2. E. Shrimpton-Phoenix, J. B. O. Mitchell, M. Bühl, Chem. Eur. J. 2022, 28, e202201728.
2.45 Kakali Sen, STFC – Modelling enzyme reactivity with QM/MM simulations
Copper nitrite reductases are enzymes occurring in a wide range of bacteria and fungi and perform a vital role in the denitrification pathway of the nitrogen cycle. The functional core of these enzymes consists of two different copper sites, one of which is involved in electron transfer and one that is the catalytic site where nitrite binds and reduction to nitric oxide occurs. The recently developed multiple structures from one crystal (MSOX) serial crystallography method can be used to provide multiple snapshots of the progress of the reduction reaction as it takes place in a protein crystal [1]. These snapshots can be used as a reference for combined quantum mechanical/molecular mechanical (QM/MM) simulations of enzyme reactivity within the crystal, which can be used to identify details of reference states that cannot be directly observed by X-ray diffraction experiments, such as protonation and oxidation states, and identify preferred reaction paths. Through a combination of MSOX experiments and QM/MM calculations we propose a mechanism for the reduction reaction in Achromobacter cycloclastes copper nitrite reductase, starting from the initial binding of nitrite to the final NO-bound structure [2]. The results are compared with QM/MM simulations performed in a solvated environment.
[1] S. Horrell, S. V. Antonyuk, R. R. Eady, S. S. Hasnain, M. A. Hough and R. W. Strange, IUCrJ 3, 271 (2016).
[2] K. Sen, M.A. Hough, R.W. Strange, C. Yong and T.W. Keal, J. Phys. Chem. B 125, 9102−9114 (2021).
3.05 Xingfan Zhang, UCL – Combining QM/MM with other Theoretical Approaches for A Comprehensive Understanding of CeO2
We combined polarisable-shell-model (PSM)-based Mott-Littleton defect calculations, electrostatic analysis, hybrid quantum mechanics/molecular mechanics (QM/MM) embedded-cluster approaches, and plane-wave DFT calculations in developing a thorough understanding of several properties of CeO2. PSM interatomic potentials are widely used for modelling charged defects in solids. However, at the pure MM level of theory, the calculated defect energetics may not satisfy the requirement of quantitative predictions and are usually limited to certain charged states. We proposed a strategy that employs accurate ionic polarisabilities, defect structures, and formation energies calculated by the QM/MM approach in developing a robust PSM potential for CeO2.1 The new potential not only reproduces a wide range of physical properties, but also unifies the predictions of intrinsic charged defects based on the MM Mott–Littleton approach and QM/MM calculations.
The ionisation potential (IP), which is the energy required to remove an electron from a solid, provides valuable information about the electronic, optical, and transport properties. While molecules have well-defined IPs, assessing the absolute IP of solid-state materials is much more challenging. CeO2 is an exceptionally interesting case where previous experiments observed significant differences in the IP ranging from 5.5 eV to 9.1 eV. To understand the origin, we employed several theoretical approaches to separate the bulk and surface contributions to the IP of CeO2. Using the QM/MM approach with complete cancellation of surface effects, we determined a theoretical bulk IP of only 5.38 eV for CeO2. Changing surface orientations can vary the IP of CeO2 from 4.2 eV to 8.2 eV, as revealed by plane-wave DFT calculations and PSM-based electrostatic analysis. These conclusions were further extended to other high-dielectric-constant MO2-type oxides such as TiO2, ZrO2, and HfO2, bridging the gap between theory and experiment. Finally, a relationship was built to correlate bulk and surface contributions to the IP with cation properties in metal oxides.
References:
1. X. Zhang, L. Zhu, Q. Hou, J. Guan, Y. Lu, T. W. Keal, J. Buckeridge, C. R. A. Catlow and A. A. Sokol, Chem. Mater., 2023, 35, 207-227.
3.25 Keith Butler, QMUL – Scratching the surface: atomistic modelling, chemical heuristics and machine learning for designing interfaces in energy materials
Materials for energy-related applications, which are crucial for a sustainable energy economy, rely on combining materials that form complex heterogenous interfaces. Simultaneously, progress in computational materials science for describing complex interfaces is critical for improving the understanding and performance of energy materials. In this presentation I will give an overview of computational approaches for understanding and tailoring interfaces for renewable energy applications. Density functional theory (DFT) has been a crucial tool for understanding the atomic and electronic structure of surfaces and interfaces and I will show how insights from DFT calculations have allowed us to propose new designs to tailor interfaces for bespoke applications. Computational screening also offers the possibility of virtual selection of materials where optimal pairs satisfy different criteria, I will present a scheme that allows rapid searching of known materials to identify mechanically stable and electronically optimal interfaces for photovoltaics. One of the limiting factors for virtual screening of interfaces is the lack of data on electronic energy levels. Recent advances in machine learning promise the ability to predict properties in a fraction of the time required for DFT calculations, thereby facilitating virtual screening, but the lack of reliable data hinders the training of such models. I will consider two approaches to overcoming this problem, first using simple chemical heuristics to estimate energy levels and second developing active learning techniques that can facilitate the generation of sparse yet representative databases of interface properties.
3.45 Tea
4.15 Thomas Keal, STFC/UCL – Scaling up computational chemistry: from small molecules to complex systems
As computers grow more powerful, they become ever more important tools for understanding chemistry. But solving the underlying mathematical equations that govern chemical processes is a struggle for even the world’s largest supercomputers. This lecture will give a personal perspective on some of the challenges we encounter when we try to simulate chemistry, and how in practice we can balance a desire for accuracy with the reality of limited computational resources.
Beginning in the realm of small molecules, we see how the properties of even the simplest chemical systems can be difficult to calculate, and how “one weird trick” makes a big difference for calculating the magnetic properties relevant to nuclear magnetic resonance spectroscopy. Next, we see how interactions with light make the challenge of simulating chemistry still more daunting, and how in practice we can model the ultrafast chemical processes that detect light in the eye.
Complex chemical systems provide a special challenge for simulation, as calculations rapidly become prohibitively expensive as the size of the model increases. In order to simulate chemistry in realistic environments, we develop methods that divide complex systems into regions which are calculated at different levels of approximation. The power and flexibility of these “multiscale” methods is illustrated with applications including the surfaces that can transform inert greenhouse gases into activated reactants, the use of spectroscopy to fingerprint gas-sensing proteins, and porous materials that can remove harmful nitrogen oxide compounds from diesel exhaust fumes.
Finally, future directions for modelling complex chemical systems will be considered, including combined computational and experimental techniques for enzyme engineering, advanced modelling methods for designing new forms of catalysts, the promise of the exascale computing era for chemical simulation, and the potential for computational chemistry to be a “killer app” for quantum computing.
5.15 Reception – Nyholm Room
Organised by:
Professor Sir Richard Catlow
tyc-administrator@ucl.ac.uk